Transient Optics & Plasmonics
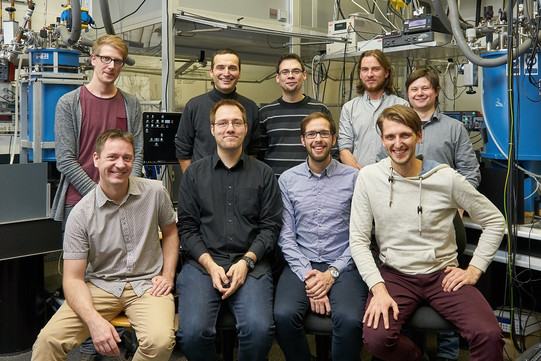
From left to right. Top row: Lars Klompmaker, Ilya Akimov, Lars Kreilkamp, Manuel Jäckl, Markus Kuhnert. Bottom row: Sergei Poltavtcev, Jonas Vondran, Felix Spitzer, Matthias Salewski.
Description of the Research
Transient optical phenomena provide essential information on dynamical properties of charge and spin states in condensed matter. This includes quantum mechanical evolution of optically prepared electronic states, their decoherence and relaxation processes. It delivers rich information on the energy structure and the main mechanisms responsible for the relaxation in the system under study. Interaction between the different fundamental quasi-particles like excitons, magnons, plasmons and phonons is especially interesting. For this purpose a new kind of hybrid materials, which combine semiconductors with excellent optical properties, ferromagnetic materials with large magnetization and metalic nanostructures with strong localization of electromagnetic field, are studied. Optical techniques like ultrafast pump probe, transient four-wave mixing in combination with magnetic field and polarization sensitive excitation and detection provide a powerful set of methods that allow to perform experimental investigation of the above mentioned phenomena. In what follows, we develop research in the following directions:
- Photon echoes in semiconductor nanostructures
- Spin interactions in semiconductors doped with magnetic impurities (GaMnAs)
- Plasmonics in combination with magnetic materials
- Exciton-polariton propagation in semiconductors
1. Photon echoes in semiconductor nanostructures
Description
Coherent optical phenomena in atomic ensembles and other systems with discrete energy level structure attract a lot of attention for the realization of optical quantum memories. One of these phenomena is the photon echo (PE) where in the classical picture an intense optical pulse (2) results in rephasing and retrieval of the macroscopic medium’s polarization, which was created by a preceding optical pulse (1). Therefore the emergence of a photon echo is based on the presence of an inhomogenity in the studied system. The decay of the PE-amplitude with increasing delay time τ12 between the excitation and refocusing pulses allows the measurement of the optical coherence time T2.
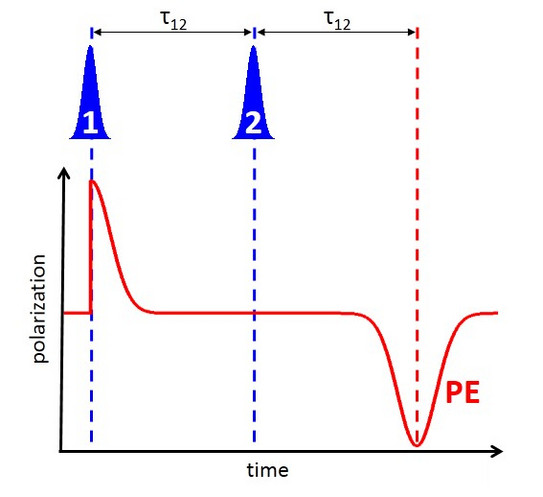
Main results
We observed photon echoes in an electron-trion system in n-type CdTe semiconductor quantum wells. The ground (electron) and the excited (trion) states are the doublets characterized by the electron (S= +1/2, −1/2) and hole (J= +3/2, −3/2) spin projections, respectively. The selection rules for optical transitions allow the coupling of the ±1/2 and ±3/2 states, respectively. An additional degree of freedom is accomplished by the application of a transverse magnetic field.
The first pulse at t=0 induces a coherent superposition of optically accessible electron and trion states (e.g. S=+1/2, J=+3/2 - called optical coherence). It leads to Larmor precession of the electron spin in the ground state. Hence the optical coherence is continuously transferred into a superposition of a pair of states that is optically inaccessible (e.g. S=−1/2, J=+3/2 - called dark coherence) and back with Larmor precession frequency. This results in an oscillatory behavior of the photon echo.
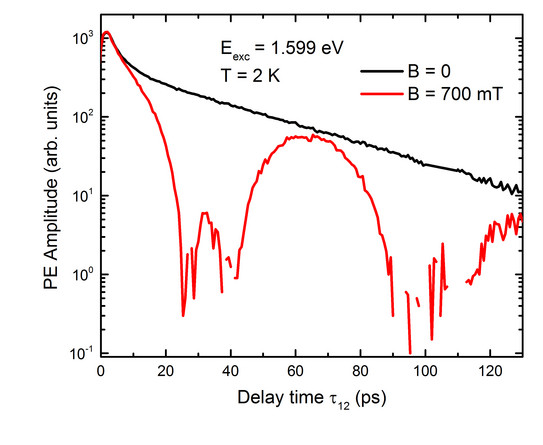
2. Spin interactions in semiconductors doped with magnetic impurities (GaMnAs)
Description
A pathway to optical spin manipulation in semiconductors is to implement magnetic ions in and exploit the strong exchange interaction between an electron (or hole) and a magnetic ion (for example Mn). Recent investigations of electron spin dynamics in GaAs doped with Mn acceptors of low concentration showed long lived electron spin memory (~20ns) and existence of optical orientation of ionized Mn acceptors in weak longitudinal magnetic fields, which are prerequisites for optical spin control. Here, the goal is to investigate various spin scenarios, e.g. non-exponential spin decay and spin precession in the effective exchange fields, which are provided by the interaction between the electrons and Mn acceptors. Spin dynamics and optical spin control of ionized and neutral Mn acceptors are of special interest. We use the combination of time-resolved photoluminescence (TRPL) and Kerr rotation, transient four-wave mixing (TFWM), as well as spin flip Raman scattering. This allows to monitor the time evolution of both electron and Mn spins. The measurements are performed in low dimensional semiconductor structures based on (In,Ga,Al)As compounds and in bulk GaAs.
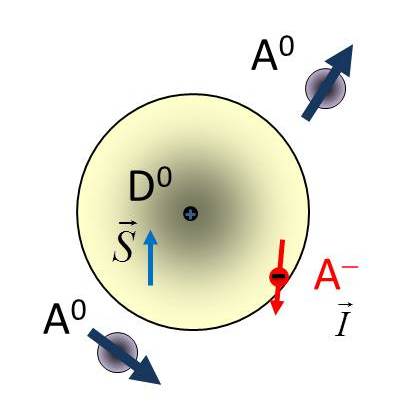
Main results
GaAs is doped with Mn acceptors (Bohr radius 1nm) of low concentration NMn~1017-1018 cm-3. In this case the material is paramagnetic since the Mn acceptors (A0) are isolated from each other. The samples are partially compensated by residual shallow donors (D0) with a concentration of about ND ~ 1016cm-3. This results in ionization of the acceptors (A-) located in the vicinity of donors if not illuminated by light. Under weak optical excitation photoexcited electrons are localized at the donors with a Bohr radius of about 10nm as it is shown in Fig.1.1. The electron spin S can be oriented by optical means using circularly polarized excitation. In the next stage s-d exchange interaction between photoexcited electrons and Mn ions provides the channel for the spin transfer into the Mn system.
Electron spin relaxation in GaAs doped with Mn
Donor-acceptor photoluminescence (PL) e-A0 corresponding to the recombination of localized electrons with the holes bound to Mn-acceptors is a direct probe of population and spin dynamics. The total PL intensity is related to the population decay I(t) = I(0) exp(-t/τ), while the degree of circular polarization ρc(t) = ρc(0)exp(-t/τS) gives direct access to the spin dynamics of oriented electrons (holes). Here τ is the lifetime and τS is the relaxation time. In transverse magnetic field, the carrier g-factor can be determined from the oscillations of ρc(t) resulting from spin quantum beats.
The total intensity transients are independent on magnetic field magnitude and direction giving the lifetime τ = 110 ns. Circular polarization transients for Voigt geometry are shown in Figure 1.2. At zero magnetic field ρc(t) decays exponentially with τS =23 ns. In transverse magnetic field we detect pronounced oscillations of the circular polarization degree, which can be well fitted by ρc(t) = ρc(0)cos(ΩLt)exp(-t/τS). The dependence of the Larmor frequency ΩL on the external magnetic field, shown in the inset, gives an electron g-factor of |g| = 0.42±0.02. This allows us to attribute the long spin memory of 23 ns to optically oriented electrons. We observe no evidence of a Mn acceptor spin orientation in zero or transverse magnetic fields.
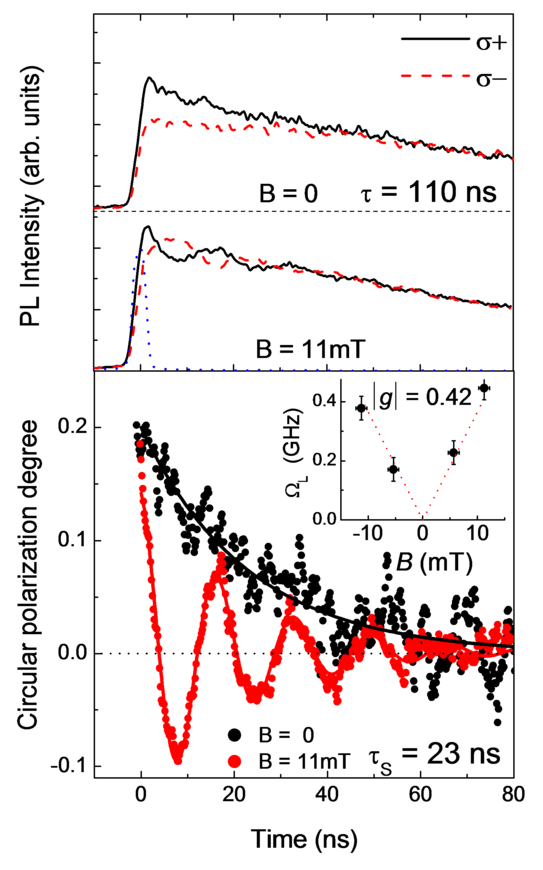
The same value for the g-factor of the electron and its spin lifetime have been measured using time-resolved Faraday rotation. Finally both experimental techniques, time-resolved Faraday rotation and TRPL spectroscopy, clearly demonstrate that in bulk GaAs doped with Mn only electrons are optically oriented, while magnetic acceptors remain unpolarized in zero and transverse magnetic fields.
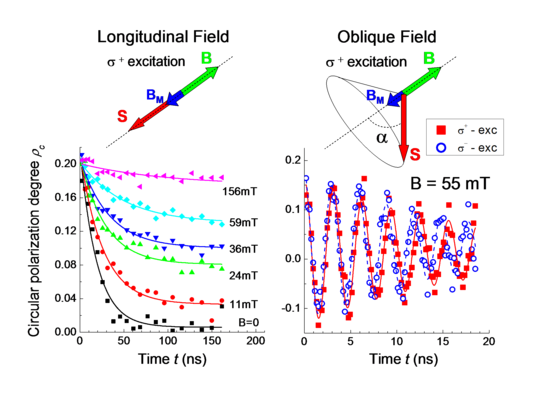
Optical orientation of Mn2+ ions in GaAs
Optical orientation of Mn2+ ions in longitudinal magnetic fields (Faraday geometry) have been observed in two types of experiments: SFRS and TRPL. In spin-flip Raman scattering (SFRS) we observe spectral lines corresponding to spin-flip processes of the ionized Mn acceptor. It follows directly from the linear magnetic field dependence of the Raman shift with Landé factor g = 2.0. The asymmetry of Stokes and anti-Stokes line intensities under circular polarized excitation allows direct evaluation of the Mn spin polarization, which can reach 25%.
TRPL in Faraday geometry shows nontrivial electron spin dynamics. At the moment of pulsed excitation the degree of polarization of the electron spins is 40%. It then decays within some tens of ns to reach a plateau. The plateau is absent at B = 0 and saturates at B = 150 mT reaching the value of 35%. Its sign changes with the helicity of incident light. It follows that s-d exchange interaction between optically oriented conduction band electrons and manganese induces a steady state non-equilibrium polarization of the Mn2+ ions. The latter is established in weak longitudinal magnetic fields, which are required to suppress the manganese spin relaxation. The optically oriented Mn2+ ions maintain the spin and return part of the polarization back to the electron spin system providing a long-lived electron spin memory. In addition the effective exchange field of manganese (up to 3 mT) can be detected via changes in the electron Larmor precession frequency in an oblique external magnetic field. This result clearly demonstrates the importance of s-d exchange interaction. The results of SFRS and TRPL agree well, supporting optical orientation of Mn ions in weak magnetic fields.
Ongoing work
We continue experimental work on optical spin control of ionized (A-) and neutral (A0) Mn acceptors in GaAs and investigation of their spin dynamics. One of the next steps is the development of various spin sensitive optical techniques like transient spin gratings (see TFWM). It should uncover the spatial and temporal evolution of both electron and Mn spins. We will also investigate low dimensional semiconductor structures based on (In,Ga,Al)As compounds and compare these studies with the bulk system.
3. Plasmonics in combination with magnetic materials
Description
The key object of plasmonics is the surface plasmon polariton (SPP) - the coupled oscillation of the electromagnetic field and the electron plasma in metals. The excitation of a SPP leads to significant electromagnetic energy localization near the metal surface and thereby enhances nonlinear effects and light-matter interaction. Current state-of-the-art criteria in telecommunications requires plasmonics to be active, i.e. a possibility for control by means of an external stimulus must be provided in the timescale of a few nanoseconds or shorter. One of the approaches satisfying the strict criteria is magnetoplasmonics employing the influence of an external magnetic field on the SPP propagation constant. While the modulation efficiency in magnetoplasmonic structures may be as large as tens of percent the operation rate is limited by the magnetization dynamics occurring on the nanoseconds timescale. Transient changes in the optical properties of plasmonic structures can be also achieved via application of intense femtosecond laser pulses.
We investigate SPPs in different types of hybrid nanostructures involving a metal layer at the top of the dielectric material being either a ferromagnet (bismuth substituted rare-earth iron garnet BiIG) or a semiconductor (GaAs, CdTe). The investigated structures are considered as plasmonic crystals because the metal film attached to the dielectric is patterned with a period comparable to the SPP wavelength. The main goals are the study of the SPP interaction with the medium’s magnetization and the establishment of different approaches for active control of SPPs.
Main results
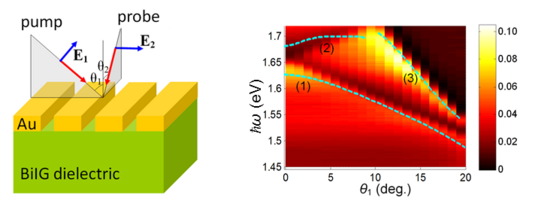
Recently we demonstrated magnetic field and optical control of SPPs in magnetoplasmonic crystals. The investigated plasmonic crystal is schematically shown in Fig. 2.1. It comprises a periodically perforated, 120-nm-thick gold film deposited on top of a smooth ferromagnetic layer of rare-earth iron-garnet BiIG with 2.5 μm thickness. The period is d = 595 nm with a groove depth of 120nm and an air groove width of 110nm (see left panel of Fig.2.1).
Magnetic field control of SPPs
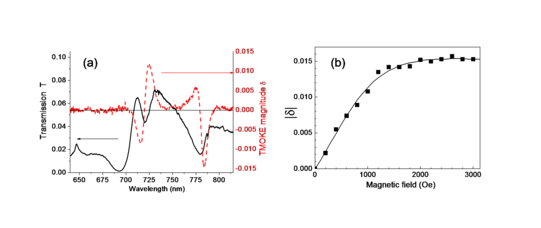
The application of a magnetic field leads to changes in the permittivity tensor of the magnetic film. As the result the propagation constant of SPP at the metal-dielectric interface is changed. Indeed experimental data shows a clear enhancement of the transverse magneto-optical Kerr effect in transmission (TMOKE) at the wavelengths of the SPP resonances related to the metal-dielectric interface (See Fig.2.2a). The changes in the propagation constant are proportional to the gyration vector component, which is parallel to the slits. This is demonstrated by the magnetic field dependence of the TMOKE signal (see Fig.2.2b).
Optical control of SPPs
Transient changes in the optical properties of plasmonic structures can be achieved via application of intense femtosecond laser pulses. Here, the real and imaginary parts of the dielectric constant of a metal change during several hundreds of femtoseconds. Since the propagation constant of SPPs is determined by the permittivity of metal and dielectric, this opens new horizons for ultrafast control of SPPs. However, the high reflectivity of smooth gold surfaces prevents most of the incident electromagnetic energy from being absorbed by the metal. Here, SPPs can be exploited to provide electromagnetic energy localization near the metal-dielectric interface and consequently to increase the energy absorption in gold.
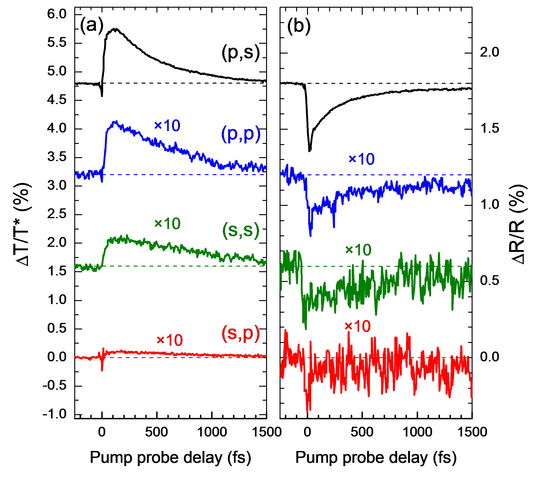
Our concept relies on time-resolved pump probe measurements, where both pump and probe interact with SPPs (see the left panel of Fig.2.1). Indeed, a pump SPP allows significant electromagnetic energy absorption in gold, whereas a probe SPP causes Fano resonances in transmission T and reflection R spectra. The latter increases the sensitivity of the differential transient signals due to the changes in the optical properties of gold. Experimental results prove this concept unambiguously (see Figure 2.3). As expected, the signals are strongest for the fully plasmonic configuration (p, s) (p- and s-polarizations of pump and probe, respectively). Compared to this configuration the signal strongly decreases for all other polarization combinations of pump and probe.
The spectral dependence of the pump-probe signal clearly demonstrates a shift of the plasmon polariton resonances with response times from 200 to 800 fs depending on the probe photon energy, by which we obtain comprehensive insight into the electron dynamics in gold.
Ongoing work
- Magnetization dynamics in magnetoplasmonic crystals under excitation of SPPs.
- Interaction between excitons and SPPs in semiconductor-plasmonic crystals.
4. Exciton-polariton propagation in semiconductors
Description
Propagation of light in semiconductors with a photon energy close to the exciton resonance in semiconductors may lead to significant slowdown of light. At the same time, excitonic polariton scattering in the lower branch is significantly suppressed due to the existence of the phonon bottle-neck. This fact can be used for long distance coherent propagation and realization of long delays for optical pulses.
Main results
We directly observed sub-nanosecond optical pulse delays in 0.75 mm thick (Cd,Zn)Te crystals, which occur during the propagation of exciton polaritons (EP) in the lower branch. The detected group velocity is only 150 times smaller than the speed of light, but the resulting time delay accumulated in the crystal can reach almost half a nanosecond. The contour plot of the transmitted laser light intensity as function of delay time and photon energy is presented in Figure 3.1. In case of spectrally narrow pulses with durations of τD=1 ps a strong dependence of pulse delay on the photon energy ħω is observed, see Fig. 3.1(a). The delay time increases when ħω approaches the exciton resonance at 1.6638 eV (vertical line). We were able to observe a maximum delay of 350 ps at ħω = 1.661 eV. For short laser pulses with τD=150 fs a strong distortion occurs, see Fig. 3.1(b). Here, the use of 12 meV spectrally broad pulses centered at 1.653 eV allows to monitor the EP dispersion in a single measurement. The spectral dependence of the group velocity is consistent with the dispersion of the lower EP branch. We were also able to detect the subsequent replicas of the pulse, which originate from reflections at the opposite crystal surfaces [see Fig. 3.1(c)]. This interesting finding demonstrates ballistic propagation of optical pulses through the crystal and indicates that EP coherence is conserved even at such long time delays.
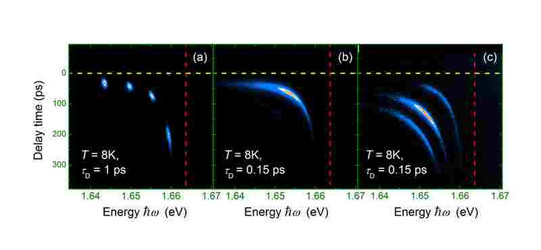
Ongoing work
The application of an external magnetic field leads to Faraday rotation and birefringence, which become much stronger in the vicinity of the resonance frequency. The polarization properties of transmitted light allow to obtain rich information on the exciton polariton energy structure, which is otherwise hidden by inhomogeneous broadening.
Current Offers For Bachelor-, Master- or PhD-Theses
Please contact us if you are interested in doing Bachelor, Master or Ph.D. on one of the topics above.
Contact
- Prof. (apl.) Dr. Ilya Akimov
- Jonas Vondran
- Lars Klompmaker
- Alexander Kosarev
- Eyüp Yalcin
Collaborations
- Laboratory of semiconductors optics, A.F. Ioffe Physico-Technical Institute, Saint Petersburg
- Spin Optics Laboratory, Saint-Petersburg State University
- Institute of Physics, Polish Academy of Sciences, Warsaw
- Laboratory of magneto-optics and plasmonics, Lomonosov Moscow State University
- Department of Physics, Paderborn University
- Dept. of Condensed Matter Physics and Material Sciences, Tata Institute of Fundamental Research, Mumbai