Optical Harmonics Generation in Semiconductors
Optical harmonics generation is associated with higher order optical susceptibilities, and it offers great potential to disclose the microscopic mechanisms of nonlinear interaction of light with matter. It is highly sensitive to symmetries and allows access to unique information about the crystallographic, electronic, and magnetic structure, which is inaccessible by linear optics. Especially, the generation of harmonics being a coherent processs creates polarized light with particular angular dependencies, and whose study and analysis provides deep insights into the involved and responsible interaction mechanisms. The most recently investigated bulk semiconductors are:
- meta-magnetic semiconductors: EuTe and EuSe
- diluted magnetic semiconductors: Cd1-xMnxTe
- diamagnetic semiconductors: ZnO, GaAs, CdTe, and Cd1-xMgxTe
- isolators: CuB2O4 and NiO
Experimental setup:
In our experiments a tunable type-II optical parametric oscillator (OPO) pumped by the third harmonic (3.49 eV / 355 nm) of a seeded solid-state Nd:YAG laser is used as a source of fundamental light in a wide spectral range from 0.6 - 6 eV. The laser system generates pulses of 8 ns duration at 10 Hz repetition rate with a typical pulse power of 15 − 60 mJ and a linewidth of about 0.5 meV. The required laser light polarization at the fundamental frequency is set by a Glan-Thomson prism and a half-wave or quater-wave plate. The resulting SHG/THG signal is analyzed by polarization optics, filtered by a double-pass spectrometer and detected by a cooled charge-coupled-device camera. The camera can also be used to picture the whole sample at once, which is useful e.g. to observe domain structures. The sample temperature can be varied from 1.2 to 300 K. External magnetic fields upto 11 T are appliable in Voigt and Faraday geometry.
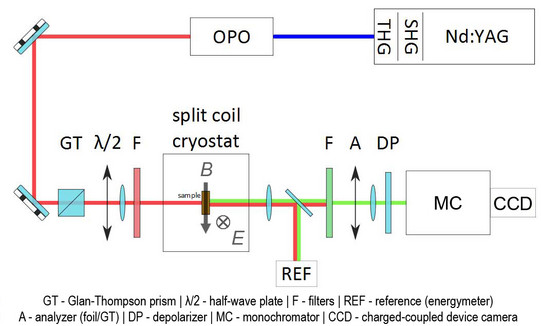
Standard equipment:
- Nd:YAG laser: Spitlight 600, Innolas
- tunable OPO: VisIR 2, GWU
- cryostat: Spectromag, Oxford Instruments
- monochromator: HR460, Jobin Yvon
- CCD camera: Pixis256E, Acton
- reference: QE12, Gentec / Rjp735, LaserProbeInc
Present focus:
The ongoing work revolves around the investigation of exciton resonances under the influence of magnetic and electric fields.
Main Results
As an overview, we suggest to read "Novel mechanisms of optical harmonics generation in semiconductors".
Mixing mechanisms of electric and magnetic fields are keys for induced resonant SHG on excitons in semiconductors
Optical second harmonic generation on excitons is studied in the wide band gap noncentrosymmetric hexagonal semiconductor ZnO in the photon energy region of 3.2−3.5 eV. Resonant crystallographic SHG is observed for 1s(A/B), 2s(A/B), 2p(A/B), and 1s(C) excitons and for an unidentified SHG resonance at 3.407 eV. Further, strong SHG signals on these exciton resonances are induced by the application of an external magnetic field perpendicular to the z-axis, even in the geometry, where the incident and SHG light wave vectors are chosen to be along the z-axis of the ZnO crystal and the crystallographic SHG is absent. The developed microscopic theory suggests several mechanisms originating from the magnetic-field-induced perturbation of the exciton structure leading to the spin Zeeman effect, the orbital Zeeman effect, the diamagnetic shift and the magneto-Stark effect. The role of each mechanism is analyzed and discussed on the basis of spectral, magnetic field, electric field, temperature and azimuthal polarization dependencies of the observed SHG signals in ZnO.
Spin polarization of Eu2+ controls optical SHG and THG in centrosymmetric magnetic EuX (X=O, S, Se, Te)
The magnetic field and temperature dependencies demonstrate that SHG and THG arises from the bulk of the materials due to a novel type of nonlinear optical susceptibility caused by the magnetic dipole contribution combined with spontaneous or induced magnetization. This spin-induced susceptibility distinctly differs from the known magnetic field induced contributions. The experimental results can be described qualitatively by a phenomenological model based on a symmetry analysis and are in good quantitative agreement with microscopic model calculations accounting for details of the electronic energy and spin structure.
Spin-splitting of electronic states essential for induced optical SHG in non-centrosymmetric diluted magnetic Cd1-xMnxTe
Depending on the Mn content in diluted magnetic semiconductor Cd1−xMnxTe (0.001≤x≤0.22, being primarily paramagnetic) magnetic field induced SHG signals arise from two distinctly different origins related to orbital and spin quantization of the band states in magnetic field. The orbital contribution dominates for zero and very low Mn concentrations, whereas the spin contribution dominates for higher Mn contents. These contributions can be distinguished by their characteristic dependencies of their SHG intensity on magnetic field. The orbital part scales as square of the field strength in accordance with a phenomenological theory. In the case of the spin quantization, the intensity scales linearly with the magnetization, a behavior which is not expected from the phenomenological model.
Orbital quantization in magnetic fields as key mechanism for induced optical SHG in non-centrosymmetric diamagnetic GaAs and CdTe
A series of narrow magnetic field induced SHG lines has been observed in the spectral range near the band gap in the III-V and II-VI diamagnetic semiconductors GaAs and CdTe. The magnetoexciton states associated with the optical transitions between Landau levels are responsible for the observed SHG spectra. Orbital quantization of electronic states is therefore established as an origin of SHG. The rotational anisotropy of the induced signal distinctly differs from that in the electric-dipole approximation. Model calculations, based on a phenomenological analysis using nonlocal contributions to the nonlinear optical susceptibility, reveal the importance of nonlinear magneto-optical spatial-dispersion, which acts together with the electric-dipole term.
Current Offers For Bachelor-, Master- or PhD-Theses
We offer Bachelor- and Master-theses in relation to the ongoing research on generation of optical harmonics by specifically breaking symmetries. Feel free to ask someone of the team for more information!
Contact
- Prof. (apl.) Dr. Dmitri Yakovlev
- Prof. Dr. em. Dietmar Fröhlich
- Andreas Farenbruch
Collaborations
-
Ioffe Physical-Technical Institute, Russian Acadamy of Sciences
-
Instituto de Física, Universidade de São Paulo
- Institut für Halbleiter- und Festkörperphysik, Johannes Kepler Universität Linz