Correlation Spectroscopy
Introduction
The behavior of indistinguishable quantum particles is governed by the spin-statistics theorem. Its best known consequence is probably the enforced antisymmetry of the wavefunction of fermions which in turn causes the Pauli exclusion principle: The probability amplitudes that lead to states where two or more fermions occupy the same state interfere destructively and thus no two fermions can occupy exactly the same state. Equally important, but less well known is the opposite effect: Indistinguishable bosons show a tendency to end up in the same state.
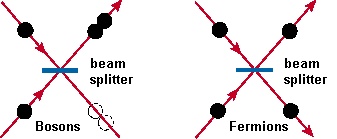
The simplest demonstration of this behavior arises for two identical particles arriving simultaneously at two different entry ports of a beam splitter. As depicted above, two bosons will always leave the beam splitter via the same exit port and two fermions will always leave the beam splitter via different exit ports. Turning that effect around allows us to draw conclusions about some particles of interest from their statistical properties. For example spontaneously emitted photons from a laser operated below threshold will show bunching behavior, while they will be emitted statistically independent of each other above the lasing threshold.
The prime quantities which determine whether particles are indistinguishable or not are the coherence volume and the coherence time. While coherence times can be pretty long for single atoms and the light emitted from them, they can be as short as a few picoseconds in semiconductor many body systems. This extremely short timescale poses a challenge for the identification of the statistical properties of light emitted from semiconductors.
Experimental approach
The most common approach to determine the statistical properties of light by using two photo diodes and performing coincidence counting is not necessarily suitable for emission processes from semiconductors as the photo diode time resolution is usually on the order of a nanosecond, while the statistical properties are visible on a timescale of tens of picoseconsds. Therefore we developed a novel approach to correlation measurements based on a modified streak camera which enables us to perform correlation measurements with a time resolution of up to 2 picoseconds. A typical picture of more than 30 consecutive emission pulses from a semiconductor laser is shown in the figure below. The upper panel gives a typical integrated streak camera image allowing us to investigate the emission dynamics. The lower panel shows photon emission events during a single emission cycle. Each white dot corresponds to a photon detection event. The insets show several photon pair detection events. Keeping track off all of these events allows us to determine the statistical properties of the emitted light. Even time-resolved and higher-order studies are possible.
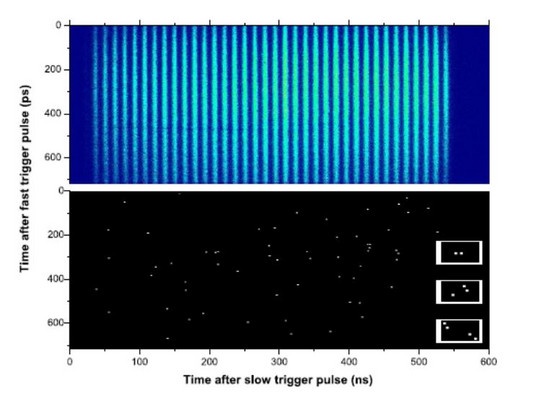
Main Results
As a first demonstration of our ultrafast streak camera correlation technique, we were able to identify the lasing threshold of quantum dot and quantum well microcavity lasers by investigating the statistical properties of the emitted light. We could show that photons emitted below the lasing threshold have a tendency to clump together. Further, we could even show that this bunching tendency gets even stronger when more photons are involved.
Details can be found in the following publications.
"Higher-Order Photon Bunching in a Semiconductor Microcavity"
M. Aßmann, F. Veit, M. Bayer, M. van der Poel, and J.M. Hvam
SCIENCE 325 (5938), 297 (2009)
DOI:10.1126/science.1174488
"Direct observation of correlations between individual photon emission events of a microcavity laser"
J. Wiersig, C. Gies, F. Jahnke, M. Aßmann, T. Berstermann, M. Bayer, C. Kistner, S. Reitzenstein, C. Schneider, S. Höfling, A. Forchel, C. Kruse, J. Kalden, and D. Hommel
NATURE 460 , 245 (2009)
DOI:10.1038/nature08126
Ongoing Work
Another well known consequence of the bosonic tendency to occupy the same state is the possibility of Bose-Einstein condensation at low temperatures. The critical temperature below which Bose-Einstein condensation can occur is determined by the mass of the condensed particles. Lighter particles allow higher condensation temperatures. For so-called exciton-polaritons, hybrid, strongly coupled quasiparticles consisting of photons and semiconductor excitations, recently condensation has been demonstrated. Owing to their light mass, about four to five orders of magnitude smaller than electrons, these could even condense at room temperature. However, they do not achieve a steady state, but show a kind of dynamical condensation. Future work will be devoted to studies of polariton condensates in optically created potentials and potential applications for all-optical switching.
Current Offers For Bachelor-, Master- or PhD-Theses
We offer Bachelor- and Master-theses in relation to the ongoing research. Feel free to ask someone of the team for more information!
Contact
- Jun.-Prof. Dr. Marc Aßmann
- Bernd Berger
- Carolin Lüders
Collaborations